Solar energy – the future of clean and sustainable powder generation. But have you ever wondered about the silent heroes behind those sleek solar panels? One such hero is a seemingly ordinary material with an extraordinary potential: tin dioxide (SnO2). Buckle up, solar enthusiasts, because we’re about to embark on a journey exploring the fascinating world of SnO2 powder and its powder to revolutionize solar cell technology.
SnO2 powder in Solar Cells: A Material with Mettle
Tin dioxide, also known as stannic oxide, is a wide-bandgap semiconductor material. Imagine a bridge connecting the world of conductors (think freely flowing electrons) and insulators (electrons firmly locked down). SnO2 sits comfortably in the middle, possessing a specific bandgap – the energy difference between its valence band (filled with electrons) and conduction band (empty and waiting for action). This bandgap plays a crucial role in its ability to convert light into electricity, a process we’ll delve into later.
But SnO2 isn’t a one-trick pony. Here’s a glimpse into its impressive portfolio:
- High Transparency: SnO2 allows a significant amount of light to pass through, a vital property for capturing the sun’s rays in solar cells.
- Excellent Electrical Conductivity: While not as good as a true conductor, SnO2 facilitates the movement of electrons within the solar cell, enabling efficient current flow.
- Stabilność chemiczna: SnO2 stands strong against harsh environmental conditions, ensuring long-lasting performance in solar applications.
- Abundant and Affordable: Tin, the source material for SnO2, is readily available, making it a cost-effective choice for large-scale solar cell production.
These combined attributes make SnO2 a highly attractive candidate for various components within a solar cell, particularly as an electron transport layer (ETL).
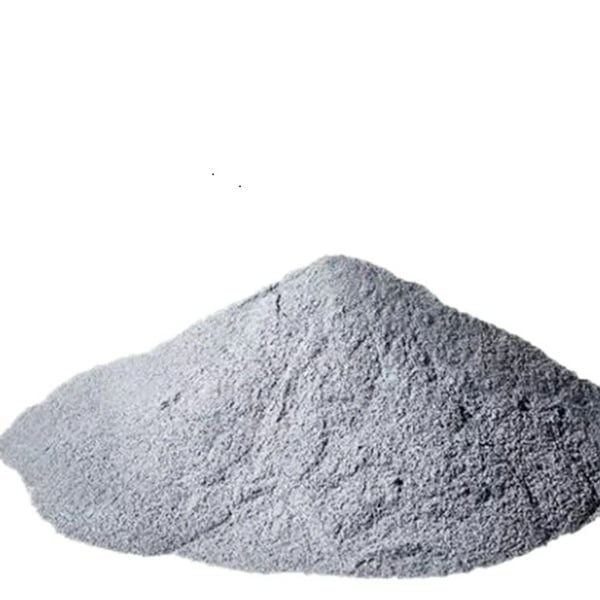
SnO2 in Solar Cells
Imagine a solar cell as a tiny powder plant. Sunlight strikes the cell, and a symphony of events unfolds. Here’s where SnO2 steps in:
- Light Absorption: Photons (light particles) from the sun hit the solar cell material (usually silicon).
- Exciton Formation: The photon’s energy excites an electron in the silicon, creating an “exciton” (an electron bound to a positively charged hole it leaves behind).
- Charge Separation: The key role of SnO2 as the ETL comes into play here. Its favorable band alignment allows the excited electron to hop across the interface between the silicon and the SnO2 layer.
- Electron Transport: Freed from the exciton, the electron merrily travels through the conductive SnO2 layer towards a collecting electrode.
- Current Generation: The collected electrons flow through an external circuit, generating electricity – voila, solar powder!
SnO2’s high transparency ensures most of the light reaches the silicon, while its excellent electron transport properties efficiently whisk away the freed electrons, minimizing recombination (electrons falling back into the holes) and maximizing powder output.
A Gallery of SnO2 powder in Solar Cells
Now, let’s get down to the nitty-gritty: the different types of SnO2 metal powders used in solar cells. Each comes with its own unique characteristics, influencing solar cell performance:
Typ | Opis | Zalety | Słabe strony |
---|---|---|---|
FTO (Fluorine-doped Tin Oxide) | The most widely used SnO2 powder. Doping with fluorine enhances conductivity. | High transparency, good conductivity, readily available. | Can be slightly expensive compared to some alternatives. |
ATO (Antimony-doped Tin Oxide) | A cost-effective alternative to FTO with similar performance. | Affordable, good balance of transparency and conductivity. | May require higher processing temperatures compared to FTO. |
Electrolytic SnO2 | Produced through an electrochemical process, resulting in highly pure SnO2 nanoparticles. | High purity, good control over particle size and morphology. | Can be more challenging to disperse uniformly in solar cell fabrication processes. |
Colloidal SnO2 | SnO2 nanoparticles suspended in a liquid medium. Offers good film-forming properties. | Easy to process, good for creating uniform thin films. | May require additional steps to remove the liquid carrier during solar cell fabrication. |
Hydrothermally Synthesized SnO2 | Produced under high pressure and temperature, leading to well-defined nanostructures. | Tailorable properties, good control over morphology and crystallinity. | Can be a more energy-intensive production process compared to others. |
P-doped SnO2 | Doping with elements like sodium or lithium creates “holes” for improved hole transport in specific solar cell architectures. | Enables p-type character for certain applications. | Can be more challenging to control doping levels precisely. |
S-doped SnO2 | Doping with sulfur can create shallow donor levels, potentially improving conductivity. | Potentially higher electron mobility compared to pristine SnO2. | May require further research to optimize doping strategies. |
Metal-coated SnO2 | SnO2 nanoparticles coated with a thin metal layer (e.g., silver) can improve conductivity and light absorption. | Enhanced conductivity, potentially improved light trapping. | Increased complexity in the fabrication process, potential cost considerations. |
Composite SnO2 Powders | Combining SnO2 with other materials (e.g., graphene) can offer synergistic properties. | Tailored properties for specific applications, potential improvements in conductivity or light scattering. | Requires careful control over composite design and fabrication. |
This table provides a snapshot of some of the most prominent SnO2 metal powders used in solar cells. The “best” choice depends on the specific solar cell architecture and desired performance characteristics. Researchers are constantly innovating and developing new SnO2-based materials to push the boundaries of solar cell efficiency.
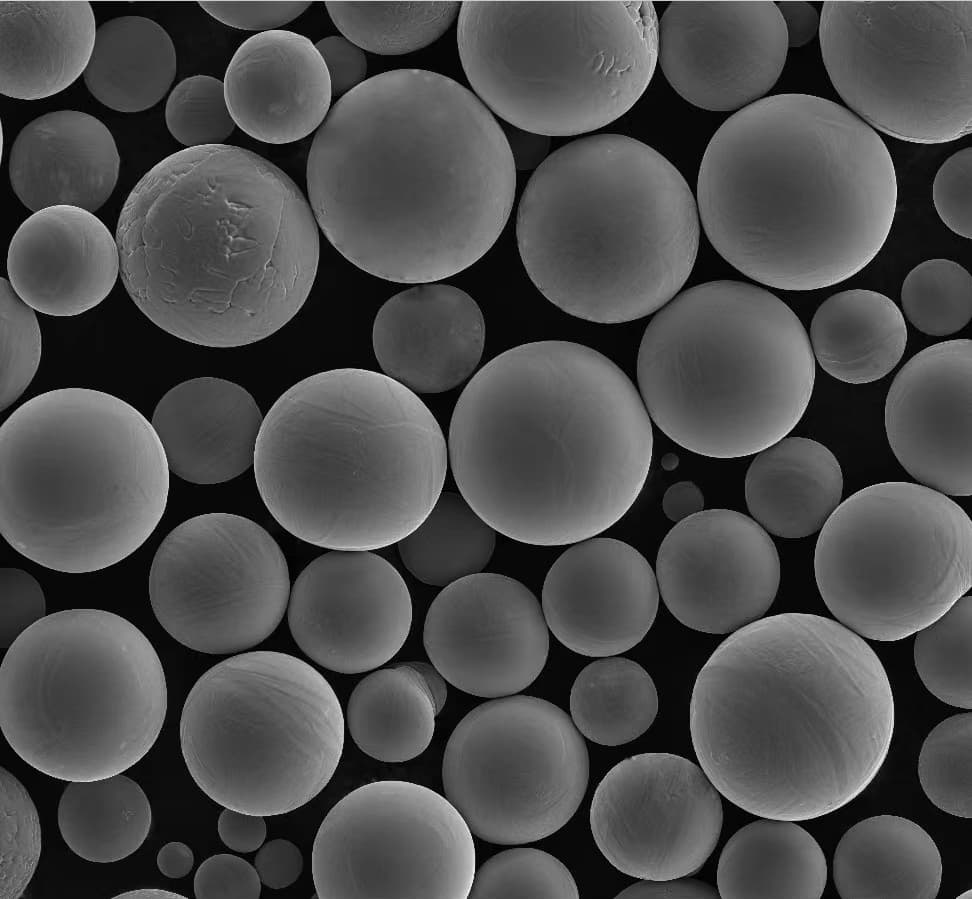
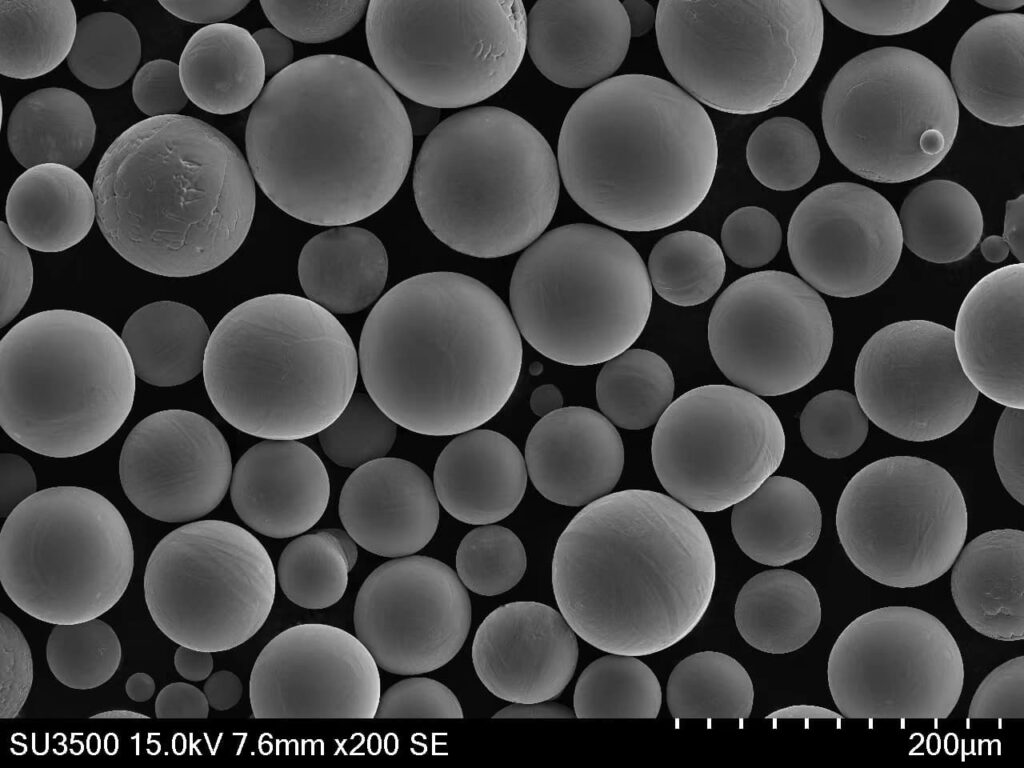
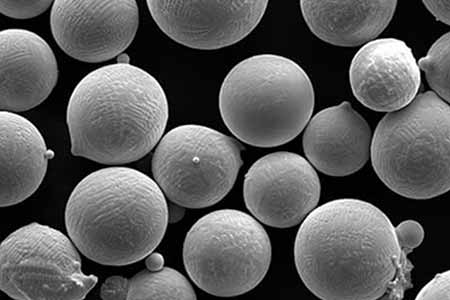
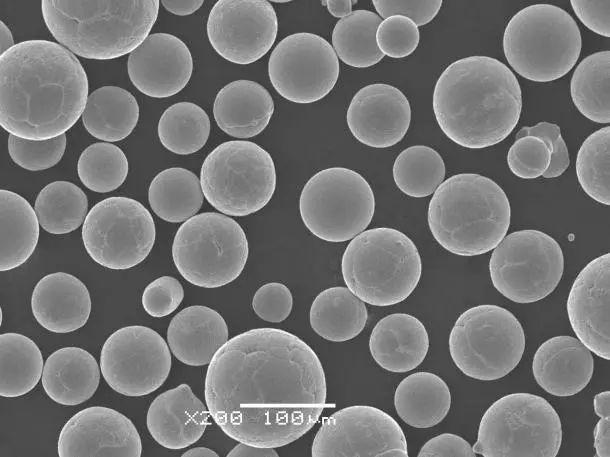
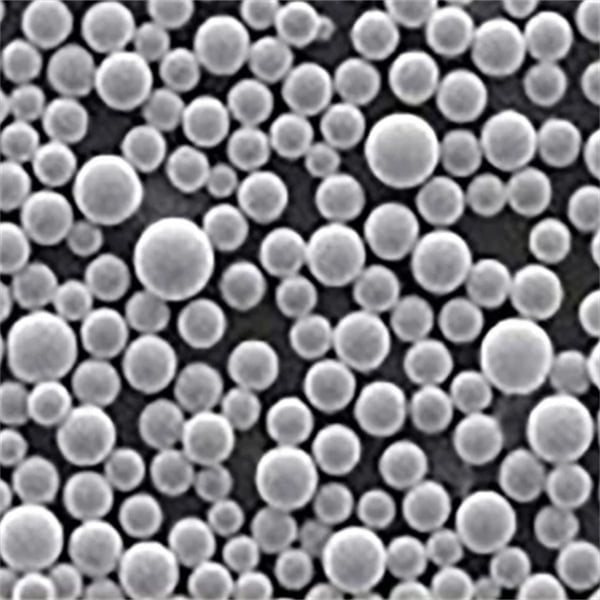
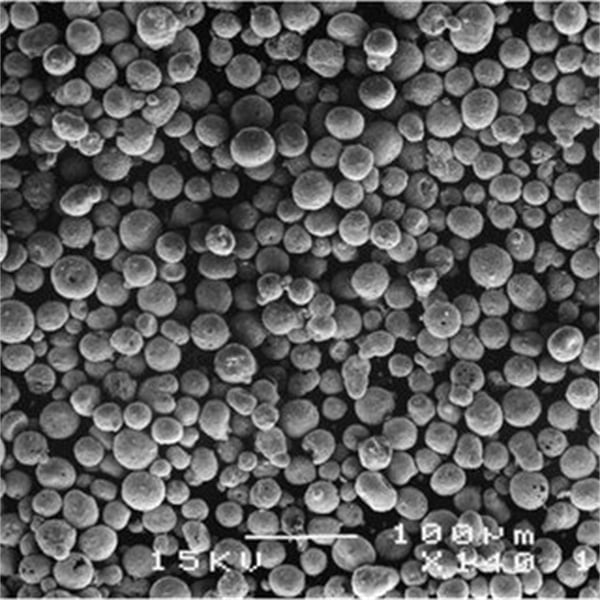
Advantages and Limitations of SnO2 powder in Solar Cells
We’ve sung the praises of SnO2, but like any good story, there are two sides to the coin. Let’s delve into the advantages and limitations of using SnO2 in solar cells:
Zalety:
- High Transparency: As mentioned earlier, SnO2 allows a significant amount of light to penetrate the solar cell, maximizing light absorption and potential powder generation.
- Excellent Electron Transport: SnO2 efficiently ferries electrons away from the silicon layer, minimizing recombination and current losses.
- Stabilność chemiczna: SnO2 stands strong against environmental factors like moisture and UV radiation, ensuring long-lasting device performance.
- Abundant and Affordable: Tin, the source material for SnO2, is readily available, making SnO2 a cost-effective choice for large-scale solar cell production.
- Tunable Properties: Doping strategies and various synthesis techniques allow researchers to tailor SnO2 properties like conductivity and bandgap for specific applications.
Ograniczenia:
- Work Function: The work function (energy needed to extract an electron) of SnO2 can sometimes create a barrier for efficient electron transfer from silicon to the ETL.
- Potential for Defects: Manufacturing processes can introduce defects into the SnO2 layer, hindering its ability to transport electrons effectively.
- Limited Light Absorption: While transparent, SnO2 itself doesn’t contribute significantly to light absorption within the solar cell.
Researchers are actively addressing these limitations. For instance, surface modifications and interface engineering techniques can help reduce the work function barrier. Additionally, advancements in synthesis methods are leading to SnO2 powders with fewer defects and improved overall performance.
Is SnO2 powder the Future of Solar Cells?
SnO2 isn’t a magic bullet, but it’s a powerful contender in the race for creating efficient and affordable solar cells. Its combination of desirable properties makes it a cornerstone material for current solar cell technologies. As research continues, further optimization and innovation promise to unlock even greater potential from SnO2. Here’s a sneak peek into what the future might hold:
- Advanced Doping Strategies: Precise control over doping levels could lead to SnO2 with even higher conductivity and tailored bandgaps for specific solar cell architectures.
- Nanocomposite Design: Combining SnO2 with other nanomaterials like graphene or metal nanoparticles could lead to enhanced light absorption, improved charge transport, and ultimately, higher solar cell efficiencies.
- Low-Temperature Processing Techniques: Developing methods to fabricate SnO2-based ETLs at lower temperatures could open doors for integration with flexible and lightweight solar cell designs.
SnO2’s journey in the world of solar cells is far from over. With continued research and development, this versatile material promises to be a key player in shaping the future of clean and sustainable energy generation.
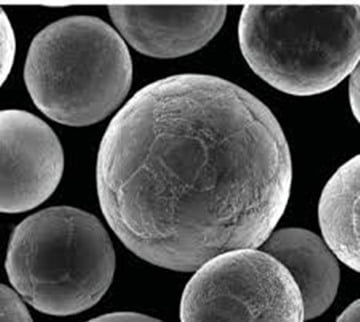
FAQ
Q: What are some alternatives to SnO2 as an ETL in solar cells?
A: Several materials are being explored as alternatives to SnO2, including zinc oxide (ZnO) and titanium dioxide (TiO2). Each offers its own set of advantages and limitations, and the choice depends on factors like desired performance characteristics and cost considerations.
Q: How is SnO2 powder applied to solar cells?
A: SnO2 powder can be applied to solar cells using various techniques, including:
- Sputtering: A physical vapor deposition method where SnO2 atoms are ejected from a target and deposited onto the substrate (silicon in the case of solar cells).
- Spin Coating: A liquid-based technique where a SnO2 precursor solution is deposited onto the substrate and then spun at high speeds to form a thin, uniform film. This film is then converted to SnO2 through a thermal annealing process.
- Spray Coating: A relatively simple and cost-effective method where a mist of SnO2 nanoparticles is sprayed onto the substrate.
The choice of deposition technique depends on factors like desired film thickness, uniformity, and overall processing cost.
Q: How can the performance of SnO2-based ETLs be improved?
A: Researchers are exploring several avenues to enhance the performance of SnO2 ETLs:
- Surface modification: Techniques like chemical treatments or atomic layer deposition can be used to modify the surface of the SnO2 layer, reducing the work function barrier and improving electron transfer efficiency.
- Interface engineering: Carefully engineering the interface between the SnO2 layer and the silicon can minimize recombination losses and improve overall device performance.
- Doping strategies: As discussed earlier, strategic doping with elements like fluorine or nitrogen can potentially enhance conductivity and tailor the bandgap of SnO2 for optimal performance.
Q: What are the environmental impacts of using SnO2 in solar cells?
A: SnO2 itself is considered a relatively benign material. However, the manufacturing process may involve the use of solvents or other chemicals that require proper disposal to minimize environmental impact. Additionally, the energy consumption associated with SnO2 production needs to be considered for a truly sustainable solar cell technology.
Informacje o 3DP mETAL
Kategoria produktu
SKONTAKTUJ SIĘ Z NAMI
Masz jakiekolwiek pytania? Wyślij nam wiadomość już teraz! Po otrzymaniu wiadomości przetworzymy Twoje zapytanie z całym zespołem.